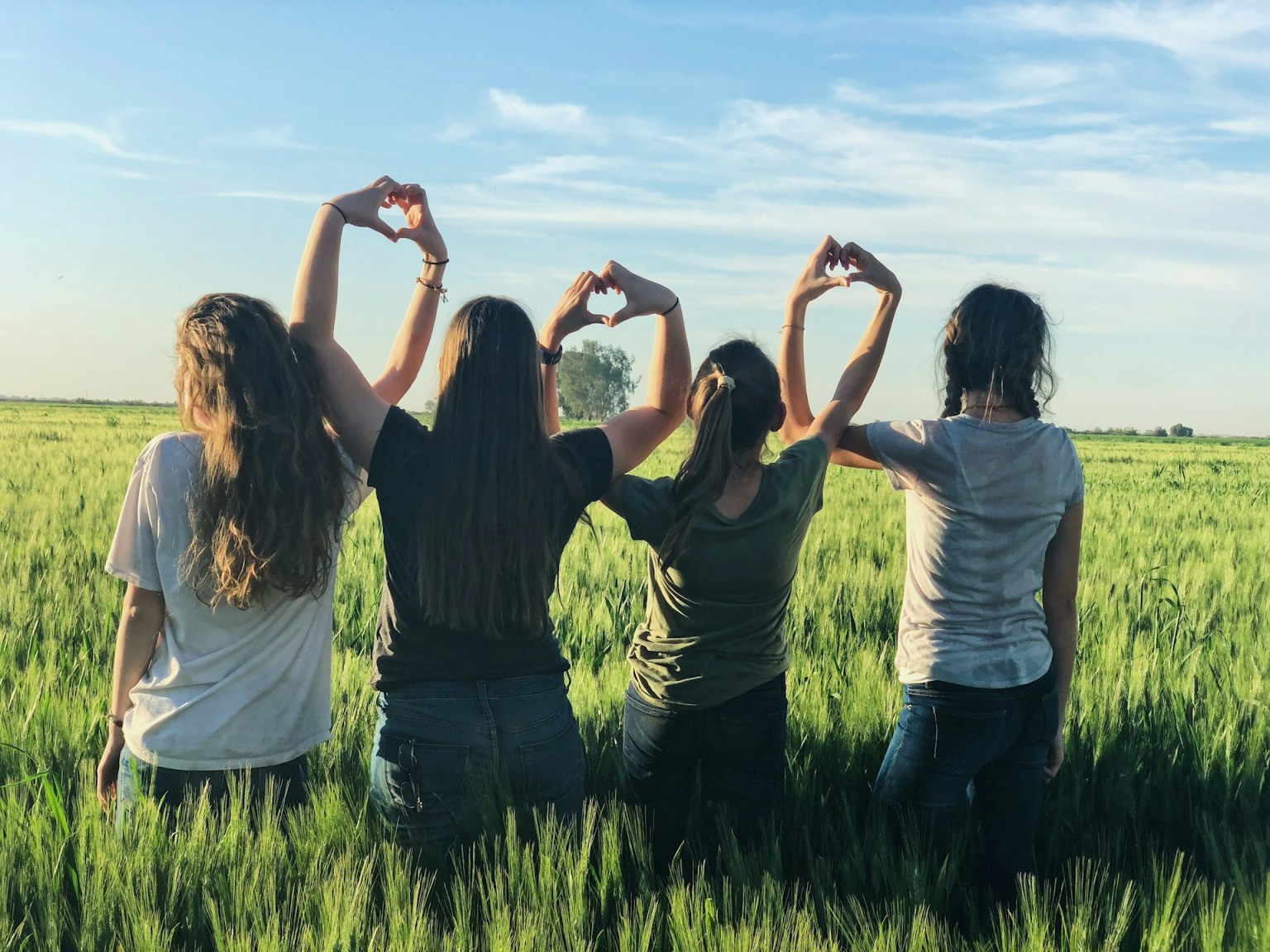
Get to know us better: Katerina Britzolaki
Kiki Barmpa
Magazine / Interviews , Science
She obtained her first degree in Chemistry from the Aristotle University of Thessaloniki in 2012, followed by a master's in Chemistry and Polymer Technology (2014) and a doctoral dissertation with the title «Development of new polymer biomaterials with simultaneous use of nano-additives and pharmaceuticals for tissue engineering applications” (2017). Since 2017, she has been working as a post-doctoral researcher in France, initially at the Sorbonne University in Paris (UPMC) and later at the Charles Sadron Institute in Strasbourg, one of the leading institutes in macromolecular Chemistry research in France. In 2018, she received a 3-year postdoctoral scholarship from the Bossodakis Foundation to work on the development of innovative nanoparticles for the simultaneous diagnosis and treatment of breast cancer.
What exactly is the subject of your research? Can you tell us what you're working on?
The research field is macromolecular chemistry, which deals with polymers or macromolecules. In my research, I am trying to exploit some of the properties of different macromolecular compounds and develop methods to diagnose and treat breast cancer. What we are trying to do in the laboratory is to synthesise magnetic nanoparticles consisting of a magnetic core and a polymeric shell that contains a chemotherapeutic agent. These hybrid nanoparticles can reveal tumours such as breast cancer via conventional Magnetic Resonance Imaging (MRI). They can also be transported within the patient's body with an external magnet. In this way, when we already know the exact tumour’s location from previous scans, we can transfer the nanoparticles to that spot and trigger the release of the drug to destroy the cancer cells once and for all.
The release of the drug from the nanoparticle happens through a process called hyperthermia. In hyperthermia, we administer intravenous preparations containing iron oxides. When an alternating magnetic field is applied, the iron oxides are forced into a rapid micro-movement, locally raising the temperature of the cell. Because cancer cells are sensitive to temperatures above 43-44 degrees Celsius, whereas healthy cells are not, cancer cells are then selectively destroyed over healthy ones. In my case, hyperthermia is used as a means of controlled release of the drug from a polymeric shell surrounding the magnetic nanoparticles. What causes the cancer cell to die is the combination of the local temperature rise with the release of the drug. So we could say that my research aims at a holistic cancer treatment by harnessing hyperthermia.
These nanoparticles are thus multifunctional since they can be used for both the treatment and diagnosis of cancer. These properties are very important because they can significantly reduce the side effects of chemotherapy, for example. Chemotherapy kills both cancer and healthy cells, which is why it has such serious side effects on patients. Hyperthermia, on the other hand, can reduce the side effects of chemotherapy through the selective targeting of cancer cells. Hyperthermia further allows an increased administered dose of the anti-cancer drug since it will mainly end up in the cancerous tumour, as well as an enhanced effect of the administered dose.
Is this process already being used as a diagnostic method? In mammograms, for example?
Sure. The ultramagnetic iron oxide nanoparticles that I study have been used for years as contrast agents in MRI for imaging the gastrointestinal tract, liver, spleen, and lymph nodes. Intravenous magnetic particles containing gadolinium, manganese, and also ultramagnetic iron oxide nanoparticles are usually administered in MRIs. These magnetic nanoparticles can be used as diagnostic tools, but they can also treat cancer by hyperthermia. Hyperthermia or oncothermia works perfectly in combination with chemotherapy, radiotherapy, and other complementary therapies. It also has wide application in sarcomas, breast and neck tumours, and local recurrences of cancer, which is why it is a widespread treatment abroad. Unfortunately, I think that in Greece, the only public hospital where it is applied free of charge is the Aretaio in Athens.
So, how do you make these nanoparticles in the laboratory? What is the process you follow?
The particles can be compared to a babushka doll, as we assemble them in successive layers. We start from the inside by chemically synthesizing the magnetic nanoparticles that will form the core. These are iron oxides, suitable for hyperthermia applications. We then synthesize a polymeric shell consisting of molecularly imprinted polymers (MIPs). While creating the polymeric shell, we use the drug molecules as a molecular 'cast'. With polymerisation, we build a polymeric network around the drug molecules and encapsulate them within it, like amber encapsulates an insect. Then, to further enhance the particle’s ability to localise cancer cells, we modify the surface of the polymeric mantle with some active targeting molecules, such as folic acid and biotin, also known as ligands. These molecules are like food to cells and make our nanoparticles even more attractive to cancer cells. The cancer cells’ tendency to proliferate at a very fast rate makes them greedy and in greater need of vitamins to proliferate.
These chemical reactions take a few days, it's not just a matter of hours. At each step of the reaction, we remove excess reagents, and at the end we characterise the final formulation by observing our products under the microscope. Then, we evaluate the physicochemical properties, the chemical structure, and the ability to release the drug in a controlled manner by applying an alternating magnetic field. We also use in vitro assays to understand the mechanism by which magnetic nanoparticles are taken up by cells under conditions that simulate possible in vivo administration. Finally, we test the particle’s cytotoxic effect on healthy and human breast cancer cells, with or without the presence of a magnetic field. The results so far are very promising, and therefore, I would very much like to soon work in vivo, testing the process on experimental animals. For the moment, however, the cost is prohibitive.
The nanoparticles do not harm the organism because although the process of phagocytosis is quite slow, the particles will eventually be broken down, and the iron they contain is released and stored in the cells as ferritin.
Is this type of therapy already being applied in some form?
Some of the elements are, individually. The use of magnetic particles and hyperthermia, for example, have been applied for years now. Targeted therapy with active targeting molecules has been patented by companies and is already being used in therapies. In contrast, the use of hyperthermia for the controlled release of a drug from a molecularly imprinted polymer is a new technique with excellent results, but still at the research level. So, although great progress has been made in the development of nanoparticles for the simultaneous diagnosis and treatment of cancer, a lot of research is still needed for these nanoparticles to reach the clinical trials phase and then some more for them to be approved by the US Food and Drug Administration (FDA). To this end, a company will have to patent the formulation and seek permission to administer it to patients after gathering all the necessary data on the safety, suitability, and biocompatibility of all the building blocks of the particles.
What happens to these particles after they have fulfilled their purpose? How are they eliminated from the body? Does it even matter, given their small size?
It depends on their size. The nanoparticles we make are about 50nm in diameter and can be eliminated from the spleen and liver through the phagocytosis system. In these organs, there are phagocytes that "surround" the "victim" particle and encapsulate it. The encapsulated nanoparticles are then stored in membrane vesicles, the endosomes, and remain there for about a month before being digested and eliminated from the body. The accumulation and agglomeration of magnetic nanoparticles in the patient's liver after months of treatment could, however, pose a problem, and this is why the safety of their in vivo administration is debated. The research group I worked with in Paris has been working a lot on this issue and has done several toxicity studies in 3D cell culture models. These studies concluded that the nanoparticles do not harm the organism because although the process of phagocytosis is quite slow, the particles will eventually be broken down, and the iron they contain is released and stored in the cells as ferritin.
Could this method be applied to types of cancer cells other than breast cancer?
Yes, of course it could. We aim to treat breast cancer, so we used the anti-cancer compound doxorubicin and binders that target breast cancer cells. Hyperthermia works very well on breast cancer indeed. In theory, however, any solid tumour lends itself to the application of hyperthermia.
Whereas you started your post-doctoral research in Paris, you later moved to the Charles Sadron Institute in Strasbourg to complete it. Why is that?
Indeed, in 2017, I started my research as a postdoctoral researcher and a Bodossakis Foundation fellow at the University of the Sorbonne in Paris. For 2 years I worked in the PHENIX laboratory as part of the research team founded by Professor Rene Massart, the first to prepare superparamagnetic magnetite nanoparticles with a simple and reproducible chemical method. This method is still considered the most successful today, and it is, of course, named after him. He is, therefore, considered, and not without reason, the "spiritual father" of these materials, and the researchers working in this laboratory have a great deal of expertise and experience in the field of magnetic nanomaterials. I sincerely believe that I couldn’t have made a better choice of research group in this specific field.
The reason now for transferring my research to Strasbourg was to be close to leading scientists again, this time in the field of polymers. Since 2019, I have been based at the Charles Sedron Institute and the group of the distinguished Professor Jean-Francois Lutz. At the Charles Sedron Institute, we combine polymers that can transport drugs with DNA molecules, resulting in materials capable of detecting cancer cells with exceptional precision. Each class of cancer cell has specific proteins on its surface, which a single chain of DNA recognizes and "locks" in the same way that the enzyme recognizes its substrate. These chains are called aptamers. They have been recently discovered and are considered the alter ego of monoclonal antibodies, only with many more advantages. Binding a synthetic polymer to a segment of a natural polymer such as DNA requires dozens of sequential reactions and is a very demanding process that we first described in an article published in the journal ACS MacroLetters.
Kiki Barmpa
Niki Michalopoulou
Danai Korre
Stella Telliou